Heavy Lift Dynamics of the Sewol Ferry Salvage
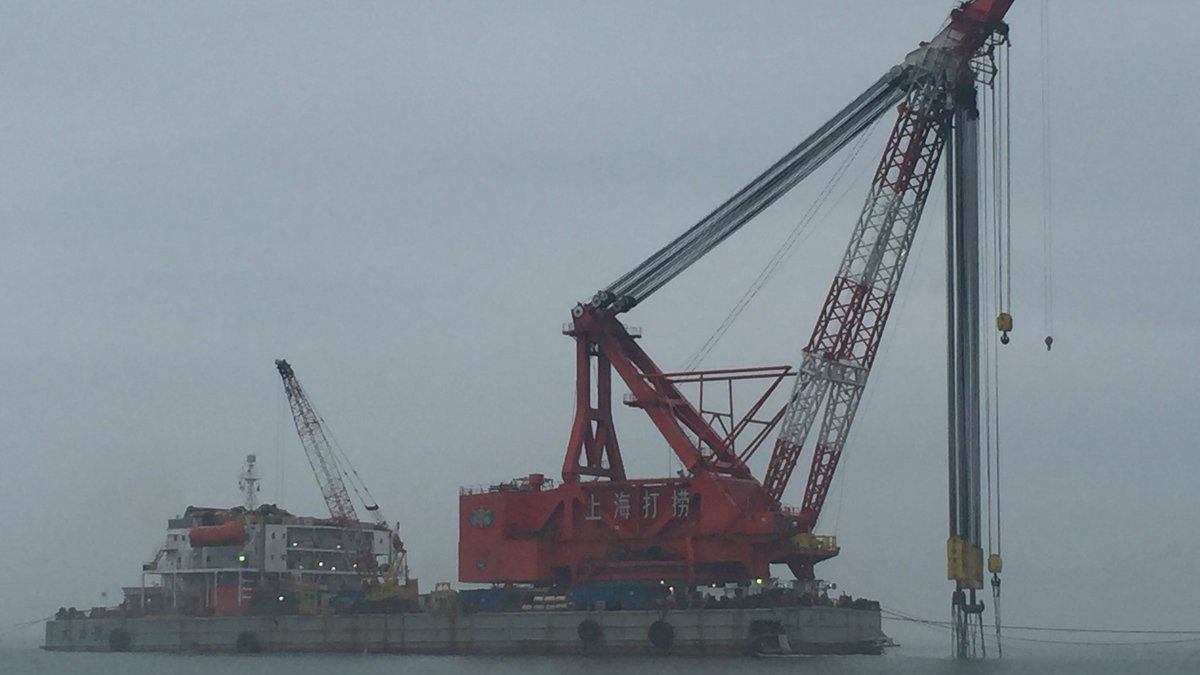
The Sewol was a RoPax vessel built in 1994. She sank off southwest Korea on April 16, 2014 with the loss of 304 lives, many of them schoolchildren. The vessel capsized and sank in 44m water depth, coming to rest on her port side on a level seabed.
Divers searched for missing bodies until November, 2015. The last body found was in October. Nine bodies were still missing at the time of writing this paper. They are believed to be inside the wreck, probably in the damaged superstructure area.
For salvage planning, OrcaFlex was used to predict dynamic sling tensions under a range of potential operating conditions. The models developed used fully coupled analysis with the motions of the Dalihao crane barge being driven by diffraction force RAOs and influenced by the crane hook loads through appropriately modeled sling systems to the submerged Sewol model. Simultaneously, the Sewol model was driven with diffraction force RAOs and was given a seabed ground reaction as well as sling loads from the crane barge. The seabed interface permitted the Sewol to respond in pitch and roll.
Salvage Stage 1 - Submerged Bow Lift
OrcaFlex Model of Bow Lift
Heel equilibrium solution during bow lift. Labels indicate vessel frame numbers. Each frame identified is drawn with a different line color in the Excel chart. The frames are shown with the vessel trimmed. Hence Frame 173, in the region where the bow slings attach, appears raised. Frames 0, 6 and 6 are indicated and can be seen to be without any significant seabed contact.
The upper short blue arrow indicates the location and magnitude of the combined sling loads. The upper red arrow indicates the location and magnitude of the combined added buoyancy force. The central purple arrow, pointing down, indicates the location and magnitude of the combined weight force. The lower brown arrow indicates the location and magnitude of FG, the ground reaction force. The variable Tpivot, is the transverse distance of FG from the keel. At this distance, FG causes a moment that balances the system such that there is no net heeling moment.
The brown trapezoidal area beneath the seabed indicates the reaction pressure that would provide the force FG, if applied over the area of hull calculated to be in contact with the seabed in this load condition.
Figure 17 (above) contains a red warning that the soil pressure diagram needs modification following the first bow lift. This is because the vessel heeled (topside going down) during the first bow lift and will have left an indented and disturbed area of soil. Heeling in the same direction would have less resistance from the soil during a second bow lift.
Numerous other data are listed on Figure 17, enabling the Excel app to be a useful tool to investigate alternative buoyancy arrangements and potential sling alternatives. For example, the soil ultimate bearing capacity used in the above results chart is 50 t/m^2.
Available ships tanks for air-filling, total useable 1,210 t
Air bags added inside starboard side on C-Deck and D-Deck, 30 t to 54 t each
External buoyancy pontoons, 500 t steel and 165t to 200t rubber
The Sewol salvage project began in 2015 and was planned to be complete by the end of 2016. This did not happen.
The main causes of delay were a failure to address the importance of heeling control during bow lifting operations and a failure to undertake a more thorough geotechnical site investigation. Secondary causes included poor attention to weather forecasts and a lack of belief that relatively large dynamic forces could be caused by quite small waves if wave periods were relatively long. A poor detail where slings passed around a deck edge resulted in a tearing failure.
Time domain fully-coupled OrcaFlex hydrodynamic dynamic analysis was used to define limiting environmental conditions for safe lifting. Limiting conditions were sling maximum tensions and Sewol buckling stresses.
Bil Stewart is the CEO of Stewart Technology Associates. He has 40 years of professional civil, structural and offshore engineering experience and is a part-time lecturer in the dynamics of offshore structures at Texas A&M University, Galveston. This article appears with his permission and may be found in its original form here.
The opinions expressed herein are the author's and not necessarily those of The Maritime Executive.